Fixed Fiber Optic Attenuators, Multimode
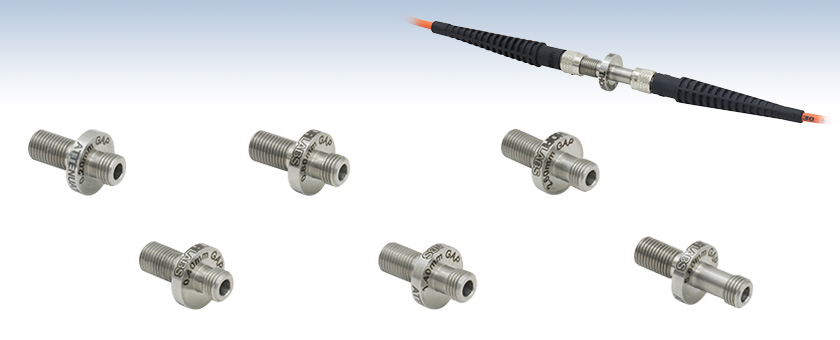
- Multimode Fixed Fiber Optic Attenuators
- Gap Lengths from 0.20 mm to 5.80 mm
- Wavelength-Independent Performance
- Compatible with SMA905 or SMA906 Connectors
FA02M
0.20 mm Gap
FA04M
0.40 mm Gap
FA08M
0.80 mm Gap
FA14M
1.40 mm Gap
FA26M
2.60 mm Gap
FA58M
5.80 mm Gap
Two M38L01 Fiber Patch Cables Plugged into an FA58M Attenuator
Application Idea

Please Wait
Fiber Optic Attenuator Selection Guide | |
---|---|
SM |
Electronic VOAs for System Integration |
Tabletop EVOAs with Power Lock | |
Manually Variable Attenuators | |
Fixed-Value Attenuators | |
MM | Fixed-Value Attenuators |
Manually Variable Attenuators | |
PM |
Electronic VOA for System Integration |
Manually Variable Attenuators |
Selection Guide | |||||
---|---|---|---|---|---|
Typical Attenuation Versus Gap Distance | |||||
Fiber NA | 0.10 | 0.22 | 0.275 | 0.39 | 0.50 |
Attenuation Plot (Click for Plot)a,b |
![]() |
![]() |
![]() |
![]() |
![]() |
Click for Raw Data |

Click to Enlarge
The SMA906-style connector's ferrule has a step, which requires using the included alignment sleeve when mating it with an SMA905-style connector.
Features
- Multimode Fixed Fiber Optic Attenuators
- Gap Lengths from 0.20 mm to 5.80 mm Available
- Wavelength-Independent Performance
- SMA905 Connectors (Alignment Sleeve for SMA906 Connectors Included)
Thorlabs' Multimode Fixed Fiber Optic Attenuators allow one to attenuate an optical signal easily by plugging multimode fibers or components directly into the attenuator. These attenuators control the attenuation by increasing the air gap distance between the two connectors, which decreases the coupling efficiency. Attenuators with fixed gap distances from 0.20 mm to 5.80 mm are available.
Item # | Nominal Gap Distancea |
---|---|
ADASMA | 0.056 ± 0.046 mm |
FA02M | 0.234 ± 0.046 mm |
FA04M | 0.437 ± 0.046 mm |
FA08M | 0.843 ± 0.046 mm |
FA14M | 1.453 ± 0.046 mm |
FA26M | 2.647 ± 0.046 mm |
FA58M | 5.847 ± 0.046 mm |
The graphs in the table to the right provide typical attenuation curves for Thorlabs' multimode patch cables with core diameters from 10 µm to 1500 µm and either 0.10, 0.22, 0.275, 0.39, or 0.50 NA. The attenuators are intended for use with two SMA-terminated multimode fibers of the same fiber type; using fibers with differing NA and core size or single mode fibers may lead to reduced performance.
Please note that the attenuation will vary depending on the fiber concentricity and SMA connector height (see the table to the right). The 10125HG SMA Height Gauge can be used to measure the height of an SMA905 ferrule. Some minor adjustment of the gap distance is possible by not completely tightening the SMA connectors on the attenuator; this can only lengthen the gap distance and cannot shorten it beyond the specified gap distance.
These attenuators are directly compatible with SMA905-style connectors, and one ceramic alignment sleeve is included for mating an SMA905-style connector to an SMA906-style connector. They are not intended for mating an SMA906-style connector to another SMA906-style connector. A washer and nut are included for panel mounting, as well as two dust caps for protecting the exposed ends of the mating sleeve. Our CAPN1 Metal Cap, sold separately, also protects the mating sleeve ends and can clip on to our L-bracket mounts.
Thorlabs also offers a variable optical attenuator for FC/PC multimode patch cables. Our multimode fiber optic filter/attenuator mount is capable of holding a variety of filters and an shutter-based attenuator; it is also designed for SMA patch cables.

Click to Enlarge
Total Internal Reflection in an Optical Fiber
Guiding Light in an Optical Fiber
Optical fibers are part of a broader class of optical components known as waveguides that utilize total internal reflection (TIR) in order to confine and guide light within a solid or liquid structure. Optical fibers, in particular, are used in numerous applications; common examples include telecommunications, spectroscopy, illumination, and sensors.
One of the more common glass (silica) optical fibers uses a structure known as a step-index fiber, which is shown in the image to the right. Step-index fibers have an inner core made from a material with a refractive index that is higher than the surrounding cladding layer. Within the fiber, a critical angle of incidence exists such that light will reflect off the core/cladding interface rather than refract into the surrounding medium. To fulfill the conditions for TIR in the fiber, the angle of incidence of light launched into the fiber must be less than a certain angle, which is defined as the acceptance angle, θacc. Snell's law can be used to calculate this angle:
where ncore is the refractive index of the fiber core, nclad is the refractive index of the fiber cladding, n is the refractive index of the outside medium, θcrit is the critical angle, and θacc is the acceptance half-angle of the fiber. The numerical aperture (NA) is a dimensionless quantity used by fiber manufacturers to specify the acceptance angle of an optical fiber and is defined as:
In step-index fibers with a large core (multimode), the NA can be calculated directly using this equation. The NA can also be determined experimentally by tracing the far-field beam profile and measuring the angle between the center of the beam and the point at which the beam intensity is 5% of the maximum; however, calculating the NA directly provides the most accurate value.
Number of Modes in an Optical Fiber
Each potential path that light propagates through in an optical fiber is known as a guided mode of the fiber. Depending on the physical dimensions of the core/cladding regions, refractive index, and wavelength, anything from one to thousands of modes can be supported within a single optical fiber. The two most commonly manufactured variants are single mode fiber (which supports a single guided mode) and multimode fiber (which supports a large number of guided modes). In a multimode fiber, lower-order modes tend to confine light spatially in the core of the fiber; higher-order modes, on the other hand, tend to confine light spatially near the core/cladding interface.
Using a few simple calculations, it is possible to estimate the number of modes (single mode or multimode) supported by an optical fiber. The normalized optical frequency, also known as the V-number, is a dimensionless quantity that is proportional to the free space optical frequency but is normalized to guiding properties of an optical fiber. The V-number is defined as:
where V is the normalized frequency (V-number), a is the fiber core radius, and λ is the free space wavelength. Multimode fibers have very large V-numbers; for example, a Ø50 µm core, 0.39 NA multimode fiber at a wavelength of 1.5 µm has a V-number of 40.8.
For multimode fiber, which has a large V-number, the number of modes supported is approximated using the following relationship.
In the example above of the Ø50 µm core, 0.39 NA multimode fiber, it supports approximately 832 different guided modes that can all travel simultaneously through the fiber.
Single mode fibers are defined with a V-number cut-off of V < 2.405, which represents the point at which light is coupled only into the fiber's fundamental mode. To meet this condition, a single mode fiber has a much smaller core size and NA compared to a multimode fiber at the same wavelength. One example of this, SMF-28 Ultra single mode fiber, has a nominal NA of 0.14 and an Ø8.2 µm core at 1550 nm, which results in a V-number of 2.404.

Click to Enlarge
Attenuation Due to Macrobend Loss

Click to Enlarge
Attenuation Due to Microbend Loss

Click to Enlarge
Beam profile measurement of FT200EMT multimode fiber and a former generation M565F1 LED (replaced by the M565F3) showing light guided in the cladding rather than the core of the fiber.
Sources of Attenuation
Loss within an optical fiber, also referred to as attenuation, is characterized and quantified in order to predict the total transmitted power lost within a fiber optic setup. The sources of these losses are typically wavelength dependent and range from the material used in the fiber itself to bending of the fiber. Common sources of attenuation are detailed below:
Absorption
Because light in a standard optical fiber is guided via a solid material, there are losses due to absorption as light propagates through the fiber. Standard fibers are manufactured using fused silica and are optimized for transmission from 1300 nm to 1550 nm. At longer wavelengths (>2000 nm), multi-phonon interactions in fused silica cause significant absorption. Fluoride glasses such as ZrF4 and InF3 are used in manufacturing Mid-IR optical fibers primarily because they exhibit lower loss at these wavelengths. ZrF4 and InF3 fibers have a multi-phonon edge of ~3.6 µm and ~4.6 µm, respectively.
Contaminants in the fiber also contribute to the absorption loss. One example of an undesired impurity is water molecules that are trapped in the glass of the optical fiber, which will absorb light around 1300 nm and 2.94 µm. Since telecom signals and some lasers operate in that same region, any water molecules present in the fiber will attenuate the signal significantly.
The concentration of ions in the fiber glass is often controlled by manufacturers to tune the transmission/attenuation properties of a fiber. For example, hydroxyl ions (OH-) are naturally present in silica and absorb light in the NIR-IR spectrum. Therefore, fibers with low-OH content are preferred for transmission at telecom wavelengths. On the other hand, fibers with high-OH content typically exhibit increased transmission at UV wavelengths and thus may be preferred by users interested in applications such as fluorescence or UV-VIS spectroscopy.
Scattering
For the majority of fiber optics applications, light scattering is a source of loss that occurs when light encounters a change in the refractive index of the medium. These changes can be extrinsic, caused by impurities, particulates, or bubbles; or intrinsic, caused by fluctuations in the glass density, composition, or phase state. Scattering is inversely related to the wavelength of light, so scattering loss becomes significant at shorter wavelengths such as the UV or blue regions of the spectrum. Using proper fiber cleaning, handling, and storage procedures may minimize the presence of impurities on tips of fibers that cause large scattering losses.
Bending Loss
Losses that occur due to changes in the external and internal geometry of an optical fiber are known as bending loss. These are usually separated into two categories: macrobending loss and microbending loss.
Macrobend loss is typically associated with the physical bending of an optical fiber; for example, rolling it in a tight coil. As shown in the image to the right, guided light is spatially distributed within the core and cladding regions of the fiber. When a fiber is bent at a radius, light near the outer radius of the bend cannot maintain the same spatial mode profile without exceeding the speed of light. Instead, the energy is lost to the surroundings as radiation. For a large bend radius, the losses associated with bending are small; however, at bend radii smaller than the recommended bend radius of a fiber, bend losses become very significant. For short periods of time, optical fibers can be operated at a small bend radius; however, for long-term storage, the bend radius should be larger than the recommended value. Use proper storage conditions (temperature and bend radius) to reduce the likelihood of permanently damaging the fiber; the FSR1 Fiber Storage Reel is designed to minimize high bend loss.
Microbend loss arises from changes in the internal geometry of the fiber, particularly the core and cladding layers. These random variations (i.e., bumps) in the fiber structure disturb the conditions needed for total internal reflection, causing propagating light to couple into a non-propagating mode that leaks from the fiber (see the image to the right for details). Unlike macrobend loss, which is controlled by the bend radius, microbend loss occurs due to permanent defects in the fiber that are created during fiber manufacturing.
Cladding Modes
While most light in a multimode fiber is guided via TIR within the core of the fiber, higher-order modes that guide light within both the core and cladding layer, because of TIR at the cladding and coating/buffer interface, can also exist. This results in what is known as a cladding mode. An example of this can be seen in the beam profile measurement to the right, which shows cladding modes with a higher intensity in the cladding than in the core of the fiber. These modes can be non-propagating (i.e., they do not fulfill the conditions for TIR) or they can propagate over a significant length of fiber. Because cladding modes are typically higher-order, they are a source of loss in the presence of fiber bending and microbending defects. Cladding modes are also lost when connecting two fibers via connectors as they cannot be easily coupled between optical fibers.
Cladding modes may be undesired for some applications (e.g., launching into free space) because of their effect on the beam spatial profile. Over long fiber lengths, these modes will naturally attenuate. For short fiber lengths (<10 m), one method for removing cladding modes from a fiber is to use a mandrel wrap at a radius that removes cladding modes while keeping the desired propagating modes.
Launch Conditions
Underfilled Launch Condition
For a large multimode fiber which accepts light over a wide NA, the condition of the light (e.g., source type, beam diameter, NA) coupled into the fiber can have a significant effect on performance. An underfilled launch condition occurs when the beam diameter and NA of light at the coupling interface are smaller than the core diameter and NA of the fiber. A common example of this is launching a laser source into a large multimode fiber. As seen in the diagram and beam profile measurement below, underfilled launches tend to concentrate light spatially in the center of the fiber, filling lower-order modes preferentially over higher-order modes. As a result, they are less sensitive to macrobend losses and do not have cladding modes. The measured insertion loss for an underfilled launch tends to be lower than typical, with a higher power density in the core of the fiber.
Overfilled Launch Condition
Overfilled launches are defined by situations where the beam diameter and NA at the coupling interface are larger than the core diameter and NA of the fiber. One method to achieve this is by launching light from an LED source into a small multimode fiber. An overfilled launch completely exposes the fiber core and some of the cladding to light, enabling the filling of lower- and higher-order modes equally (as seen in the images below) and increasing the likelihood of coupling into cladding modes of the fiber. This increased percentage of higher-order modes means that overfilled fibers are more sensitive to bending loss. The measured insertion loss for an overfilled launch tends to be higher than typical, but results in an overall higher output power compared to an underfilled fiber launch.
There are advantages and disadvantages to underfilled or overfilled launch conditions, depending on the needs of the intended application. For measuring the baseline performance of a multimode fiber, Thorlabs recommends using a launch condition where the beam diameter is 70-80% of the fiber core diameter. Over short distances, an overfilled fiber has more output power; however, over long distances (>10 - 20 m) the higher-order modes that more susceptible to attenuation will disappear.
Posted Comments: | |
Alexandre Tassé
 (posted 2020-08-28 11:53:43.527) I can't find the material in which the FA08M is manufactured. Can you please let me know? Thank you.
Alexandre Tassé. YLohia
 (posted 2020-08-28 02:41:20.0) Hello Alexandre, thank you for contacting Thorlabs. The attenuator body is made of 303 Stainless Steel. This information is given in the product drawing. doyehw
 (posted 2017-05-23 15:57:45.79) The description states the attenuation is wavelength independent - are there any graphs showing this? Maybe I misunderstand how the gap works... It would be very useful to demonstrate the attenuation is wavelength independent! nbayconich
 (posted 2017-06-12 09:16:50.0) Thank you for contacting Thorlabs. These fixed fiber optic attenuators control attenuation of a signal by increasing the air gap distance between two fiber ends. The dependence on wavelength for these attenuators is negligible since the light is only travelling through air. A Techsupport representative will contact you directly. cbrideau
 (posted 2016-09-02 16:28:13.1) Would love to see an FC to SMA version of this for measuring spectra of FC sources with an SMA-fiber coupled spectrometer. user
 (posted 2016-09-02 15:06:26.747) Interesting product. I am curious if cladding mode is well blocked the attenuators? tfrisch
 (posted 2016-09-07 01:04:54.0) The attenuation is typical for an overfilled fiber, but the air gap does not block light from coupling into the cladding of the second fiber. For more details on mitigating cladding modes, please contact TechSupport@thorlabs.com |