Dichroic Atomic Vapor Spectroscopy Kit
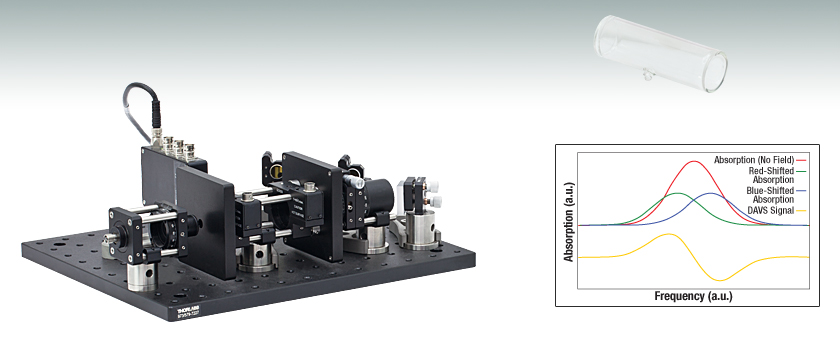
- Ideal for Laser Locking or Teaching Labs
- Rubidium or Potassium Vapor Cells Available Separately
SKDAV
See the DAV Spectroscopy Tab Below
for Measurement Details
GC25075-RB
Rubidium Vapor Cell

Please Wait
Spectroscopy Systems Selection Guide |
---|
Dichroic Atomic Vapor Spectroscopy (DAVS) Systems |
Saturated Absorption Spectroscopy (SAS) Systems |
Specifications | |
---|---|
Long-Term Stability | <2 MHz (RMS) |
Required Input Power | ~100 µW |
Input Fiber Termination* | FC/PC |
Wide Capture Range | ~500 MHz |
Detector Bandwidth | 1 MHz |
Detector Output Range | ±10 V |
Reference Cell Temperature | 50 °C (Max) |
*Alternate fiber inputs are available. Please contact techsupport@thorlabs.com.
Features
- Dichroic Atomic Vapor Spectroscopy (DAVS) for Locking to Transitions
- Rubidium, Potassium, and Custom Vapor Cells Available
- PM Fiber-Coupled Setup
- Ideal for Locking Tunable Lasers or Teaching Labs
The Thorlabs SKDAV kit consists of a proven set of components to construct a compact, fiber-coupled dichroic atomic vapor spectroscopy (DAVS) setup. It offers a method for producing a highly stable lock for tunable lasers, with a wide capture range, low power requirements, and steep signal slope. The kit also allows for the study of the Zeeman effect in atomic transitions.
The kit has been designed using stock optics and mechanics as well as compatible custom components, making it compatible with other Thorlabs cage system and lens tube components. For a list of the major components and subsystems included in the DAVS kit, please see the Kit Contents tab.
While the vapor cell heater is included in the kit, please note that the vapor cell and temperature controller must be purchased separately. Currently, we offer rubidium and potassium reference vapor cells, which are available below. A variety of custom vapor cells are also available; please contact techsupport@thorlabs.com for more details. Thorlabs' TC200 Temperature Controller is compatible with the cell heater and sold seporately below. We also offer pre-assembled Herriott cells for gas absorption spectroscopy applications.
Dichroic Atomic Vapor Spectroscopy
Dichroic Atomic Vapor Spectroscopy (DAVS) utilizes the Zeeman effect to create a signal suitable for laser locking. A rubidium vapor cell is placed in a weak longitudinal magnetic field. The Zeeman splitting of the atomic transitions allows for wavelength locking at locations off of resonance peaks. For a detailed tutorial on DAVS, please see the DAV Spectroscopy tab.
Custom Options and Assembly Services
Our spectroscopy kits are adaptable to most tunable lasers with user-supplied feedback mechanisms. While our standard kit is designed to accept PM fiber-coupled sources, we can also offer kits for free-space input. We can also offer assembly services upon request. To discuss these and other custom options, please contact us at techsupport@thorlabs.com.
DAVS Kit Contents
Thorlabs' DAVS Kit contains the following subsystems:
Fiber Input and Polarizer
The fiber input is designed with our F220FC-780 fiber collimator and cage system components. The F220FC-780 collimates the input from an FC/PC-terminated PM fiber, which must be purchased separately. For Rubidium, we recommend using our P1-780PM-FC-5 patch cable. For users who would prefer a free-space input, please contact us at techsupport@thorlabs.com for a quote for a kit without the fiber collimator and mounting mechanics.
Once the light is collimated, the beam passes through a calcite Glan-Laser polarizer to ensure polarization purity. Both the fiber collimator and polarizer are provided with our cage system mounts, which easily allows for the user to add other components using our 30 mm cage system.
Vapor Cell Heater and Magnets
This assembly consists of our GCH25-75 vapor cell heater and a permanent magnet assembly specifically designed for the DAVS kit. Both magnets feature a hole in the center to allow the light to pass, and they attach to the gas cell heater using cage system ER rods included with the heater. The magnets are easily removed so that both absorption and DAV spectra may be measured.
Please note that neither vapor cells nor a temperature controller for the GCH25-75 are included with the SKDAV. Rubidium and Potassium cells are available on the bottom of this page, and other atomic vapor cells are available upon request. Thorlabs offers the TC200 temperature controller which is compatible with the vapor cell heater.
Output Prism
After exiting the vapor cell and magnet assembly, the light will consist of two counter-rotating circular polarizations, which will be absorbed at either red- or blue-shifted frequencies (see the DAV Spectroscopy Tab for details). A zero-order quarter-wave plate (WPQ05M-780) will map the circular polarizations onto orthogonal linear polarizations. These linear polarizations are then split by a Wollaston Prism (WP10-B) at an angle of 20°.
Both polarization optics are mounted into 30 mm cage system rotation mounts (the quarter-wave plate is mounted into a CRM1P while the prism is mounted using a CRM1. The CRM1P features a micrometer that allows for very small adjustments of the wave plate, which in turn leads to finer adjustments of the locking frequency. The use of these cage system and SM1 lens tube mounts would allow easy addition of additional optics or mechanical components according to experimental needs.

Mirrors and Balanced Detector
A square folding mirror and kinematic mirror mount are included to direct the output of the Wollaston prism so that the assembly can be made more compact. If space is not an issue, this mirror can be left out of the system. After reflecting off of the square mirror, the two orthogonal polarizations are reflected separately by Ø1/2" mirrors, so that the beams can be aligned with the two inputs of our PDB210A balanced detector. The image to the right shows the beam path through the entire system, which illustrates the function of the mirrors. The balanced detector allows for measurement of three output voltages: the separate signals recorded from each photodiode, as well as the difference between the two measurement channels. This difference channel provides the DAVS signal, which can be used for a stable laser lock. The other two chanels are useful for alignment of the system, as well as to illustrate the principles of DAVS in teaching labs.
Dichroic Atomic Vapor Spectroscopy

Figure 1. In the absence of a magnetic field, the absorption profile is independent of polarization, as shown by the red line in the graph. After a longitudinal magnetic field is applied, the Zeeman shift can be observed for the two circularly polarized components (green and blue lines). The useful DAVS signal is the difference between the absorption profiles of these two components.
Introduction
Dichroic Atomic Vapor Spectroscopy utilizes the Zeeman effect to create a stable frequency lock for a laser. A vapor cell is placed in a weak longitudinal magnetic field, and linear polarized light passes through the cell. Due to the presence of the weak magnetic field, the absorption profiles of the two circularly polarized components (left and right) that comprise the linearly polarized input beam are shifted to higher and lower frequencies, respectively. After passing through the vapor cell, the beam propagates through a quarter-wave plate and a polarizing beamsplitter. A dispersion-like curve is generated, which provides an error signal for the frequency lock.
The following tutorial gives details on the basics of how DAVS spectroscopy works and how it can be utilized to lock a single-frequency laser, and the advantages of our DAVS system.
Zeeman Splitting due to a Magnetic Field
In the presence of a magnetic field, the hyperfine transitions will be split by the Zeeman effect. In the absence of a magnetic field, the absorption of an atomic vapor is independent of the polarization of the excitation source. This is shown by the red line in Figure 1. If the light is linearly polarized, it can be thought of as a superposition of counter-rotating circular polarizations (left and right).
The DAVS System
The DAVS system consists of an atomic vapor cell in a weak longitudinal magnetic field. The schematic of the system is shown in Figure 2. The input laser light travels through a Glan-Taylor polarizer prior to entering the vapor cell, ensuring that the light is linearly polarized. After propagating through the vapor cell, the beam passes through a quarter-wave plate and a Wollaston prism, which serves as a polarizing beamsplitter.
Figure 2. Schematic of the DAVS system.

Figure 3. Using DAVS, a laser can be locked to any of the zero crossings in the above signal. The circled zero crossings correspond to transitions in atomic Rb, each of which can be tuned by approximately 500 MHz.
Due to the presence of the magnetic field, the absorption profiles will be split as shown in Figure 1; the initial linear polarizer ensures that the light will be in one of the two circular polarization states to interact with the two Zeeman-split absorption curves. The quarter-wave plate will map the two circular polarization components into two orthogonal linear polarization components, which are then split by the Wollaston prism to be detected by the balanced detector. The resulting measured signal is the difference between the two Zeeman-split absorption curves, and is the yellow line shown in Figure 1.
One of the advantages of the DAVS system lies in the quarter-wave plate. If the waveplate's axes are 45° from the axis of the input Glan-Taylor polarizer, then the two absorption components will be equal in intensity after being split by the Wollaston prism. The laser is locked to the zero-crossing in the signal measured by the balanced detector. The zero crossings for the Rb D2 transition are shown in Figure 3. As the quarter-wave plate is rotated, the two absorption profiles will pass through with differing intensities; this will cause the zero-crossing point to shift; in Thorlabs' DAVS kit, each of the locking points can be tuned by up to 500 MHz, which is referred to as the capture range.
Posted Comments: | |
No Comments Posted |


Click to Enlarge
Borosilicate Glass Transmission
Thorlabs offers Potassium and Rubidium cells that are compatible with our Spectroscopy Kits. These reference cells are fabricated from borosilicate glass, a rugged material known to resist chipping and cracking. They are tested to ensure that the transmission through the cell exceeds 84% for light in the 350 nm to 2.2 µm range.
MSDS sheets are available and can be found by clicking on the red Docs icon () below.